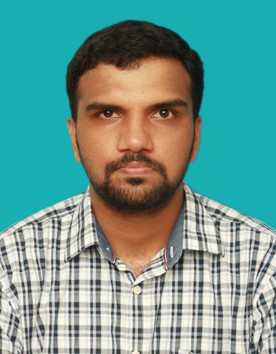
Assistant Professor
Dept. of Civil Engg.
College of Engineering, Vatakara, Kozhikode
The inherent advantages of the conventional planar reinforcing elements and the passive resistances offered by the additional members render three dimensional reinforcements, ideal for earth reinforcement. Their use is gradually gaining popularity in the field of ground improvement. This investigation aims at studying the feasibility of using plastic multi-oriented reinforcements for improving the shear strength of saturated sand fractions. A systematic and comprehensive set of drained and undrained triaxial compression tests were conducted on unreinforced and reinforced saturated sand fractions. The effect of effective grain size (d10), confining pressure (σ3) and drainage conditions on the response of saturated sand fractions, were determined by conducting parametric studies. Experimental results indicate that the addition of multi directional reinforcing elements improved the peak deviatoric stresses in sand fractions. The pore pressure dissipation improved along with a substantial reduction in peak volumetric strains.
1 INTRODUCTION
Soil reinforcement has been widely used in geotechnical engineering applications such as construction of road and railway embankments, bridge abutments, stabilization of slopes, improvement of soft ground, and so on. In the initial stages, reinforced soil typically consisted of strips, grids or sheet elements placed horizontally in the soil. Later on, reinforcement techniques such as use of continuous filaments and randomly distributed small discrete inclusions in the form of fibres, rods, discs, shavings, meshes and multioriented elements, came into the picture [1]. Numerous researchers have examined the effect of geosynthetics, in the form of membranes, fibres, cells etc. on soil [2-10]. It was found that the planar form of reinforcement in sand using geosynthetic layers changed the behaviour of soil specimen from brittle to ductile [11-13]. Randomly oriented discrete geosynthetic fibers improved the strength, stiffness and reduced the post peak loss of shear in sands [14-20]. The strength parameters of sand fractions have been found to improve by the use of three dimensional reinforcements in the form of ring reinforcements with varying heights of vertical elements [21,22]. The effects of multi-directional reinforcements on saturated sand fractions, under varying conditions of drainage are investigated in this study. Consolidated undrained and drained triaxial compression tests are performed on saturated reinforced and unreinforced coarse, medium and fine sand fractions. The effect of plastic three dimensional reinforcements on dry sand fractions have been investigated vide triaxial compression tests [23]. Coarse, medium and fine sand fractions are mixed with random and layered arrangement of reinforcing inclusions. The addition of such reinforcements improved the shear strength parameters of soil. When added in a random manner, an optimum reinforcement content of 2.8% by volume of the sand sample is arrived at, based on the improvements in strength obtained. The results obtained from this study forms the basis of the investigations carried out in the present paper.

2 EXPERIMENTAL PROCEDURE
2.1 Test materials
Clean river sand, obtained from the premises of NIT Calicut, Kerala, India was used for the study. It was sieved and separated into coarse, medium and fine fractions, in accordance with Indian Standards IS 1498:1970. Table 1 summarizes the engineering and index properties of the sand fractions. The three dimensional reinforcements were manufactured locally by injection moulding of ABS plastic. The reinforcements consisted of four legs or protrusions in a single plane (x-y) and two protrusions in plane perpendicular to this plane (z). The reinforcements measured an average length of 15 mm and diameter of 4 mm. Fig.1a shows the three dimensional reinforcements used for the study. Further properties of the reinforcing elements, as enlisted by the manufacturer are listed in Table 2.The grain size distribution of the sand fractions is shown in Fig.1b.
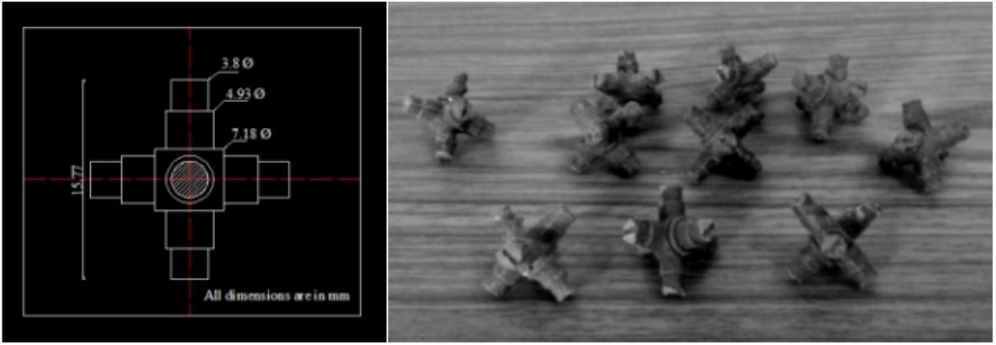
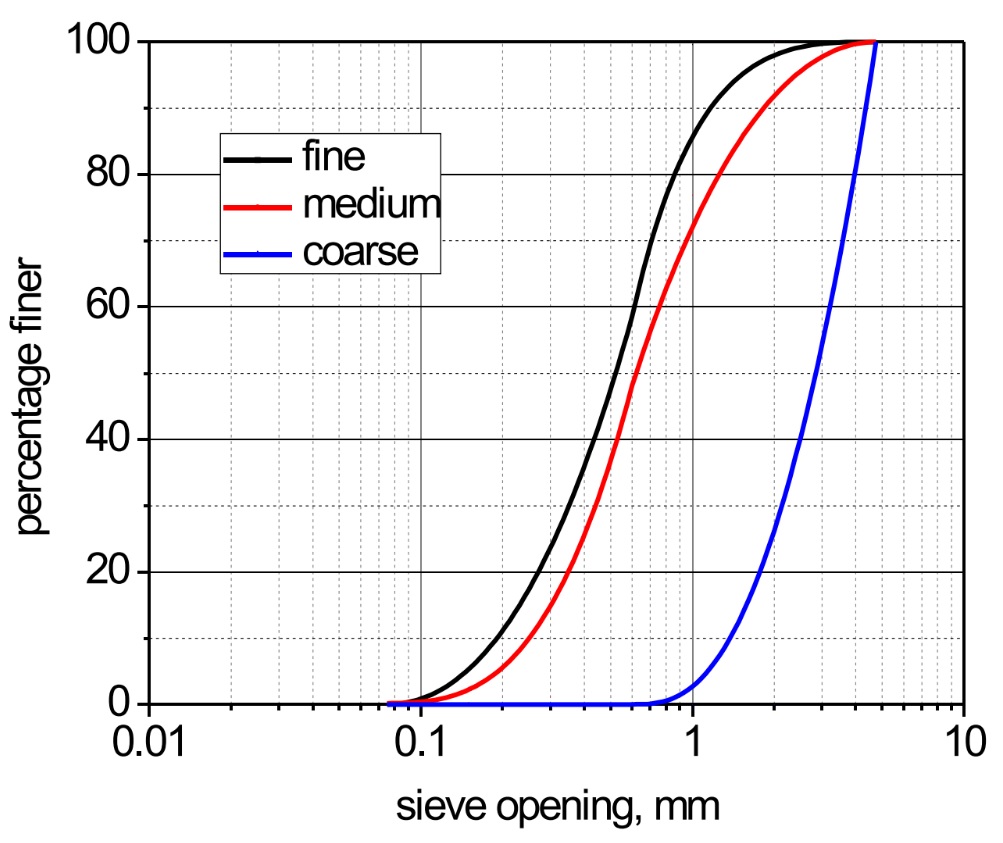
Table 1. Properties of sand fractions
IS classification | coarse | medium | fine |
Specific gravity | 2.7 | 2.72 | 2.63 |
Maximum dry density (g/cc) | 1.509 | 1.487 | 1.543 |
Minimum dry density (g/cc) | 1.355 | 1.253 | 1.319 |
D10 (mm) | 0.35 | 0.35 | 0.26 |
D50 (mm) | 1.24 | 0.61 | 0.55 |
Cu | 1.097 | 2.03 | 2.31 |
Cc | 0.977 | 0.81 | 1.025 |
Table 2. Properties of multi-directional inclusions
Material | ABS plastic |
Specific Gravity | 1.04 |
Glass transition temperature | 1050 C |
Tensile strength | 30 MPa |
Volume of 1 inclusion | 691.24 mm3 |
Mean length | 15 mm |
Mean diameter | 4 mm |
Mean surface area | 765 mm2 |
3 TEST RESULTS AND DISCUSSIONS
3.1 Consolidated Undrained tests with pore pressure measurement (CŪ)
The experimental procedure commenced with the determination of the optimum content of multi-oriented reinforcement in dry sand fractions [23]. Dry sand specimens, 50 mm in diameter and 100 mm high were prepared in a standard triaxial cell. In order to simulate medium dense conditions, a relative density of 65% was maintained. The strain rate was fixed as 0.6% per minute for the sand fractions, following standard guidelines [24,25]. Confining pressures of 100, 200, 300 and 400 kPa were applied to the specimen. Each reinforced sample was prepared to the same soil matrix density as the unreinforced case, so as to determine the actual response of sands. That is, the weight of soil required for achieving a given density was determined by subtracting the volume of the reinforcements from the total volume of the soil sample. Thus the relative density was kept constant in all cases. A series of undrained triaxial compression tests were conducted on these fractions, reinforced with 1.05, 2.11, 3.17 and 4.22 % of reinforcements, added by volume of the sand sample in the triaxial cell. These percentages correspond to 3, 6, 9 and 12 number of reinforcement inclusions respectively. The results of triaxial compression tests indicated that an optimum reinforcement content of 2.8%, or eight number of reinforcement inclusions provide the maximum increase in the peak deviatoric stress and the angle of internal friction for coarse, medium and fine sand fractions in the dry state, as shown in Fig.2. It was also concluded that a four layered reinforcement configuration imparted better results, compared to a random arrangement of reinforcements. In this study, an attempt was made to reinforce the sand fractions in saturated conditions and determine the response obtained thereof. To determine the maximum improvement to shear strength parameters, the reinforcements were added, symmetrically, in four layers, at an inter-layer spacing of 16 mm. A new technique was used for saturating the sand specimen [26]. The specimens were prepared at an initial water content of 5%, within a modified split-sand former mould. The triaxial cell pedestal along with the specimen was immersed in a water bath with a water head of about 1 m. The cell pedestal was taken out of the water bath after 24 hours and the perspex cylinder was screwed on to the cell pedestal. The region surrounding the sample was filled with water, coupled with the gradual removal of the split-sand former. This ensured minimum disturbance to the saturated sample inside the rubber membrane, once the split mould has been removed. Additionally, to verify the results, the B parameter check was also performed before the commencement of some of the tests and was obtained as 0.96, on an average. Further details of the test setup for ensuring saturation are given elsewhere [26]. Consolidated drained (CD) and undrained (CŪ) tests were performed on the sand fractions. The effect of reinforcements on the pore pressure dissipation and the volume change under conditions of varying confining pressure (σ3) and effective grain size(d10) were analysed. Volume ratio of reinforcement, Vr=Vg/Vs, where Vg is the volume of multi-directional reinforcements and Vs is the volume of the soil sample in the triaxial cell.

Strain controlled, consolidated undrained (CŪ) triaxial compression tests were performed on saturated sand fractions, both in unreinforced and reinforced conditions. The triaxial cell was suitably modified in order to ensure specimen saturation, which was followed by immersion of the sample in a water bath with sufficient water head [26]. A pore pressure transducer of 5 bar capacity recorded the instantaneous pore pressures and an LVDT measured the axial displacements in the sample. Both the pressure transducer and the LVDT were connected to a multi channel data acquisition system with computer interfacing.
3.1.1 Deviatoric stress-axial strain curves
Typical deviatoric stress-axial strain plots, for unreinforced and reinforced sand fractions are shown in Fig.2a-2b. Maximum values of peak deviatoric stresses were achieved at strain rates of 6-8% for all sand fractions. Saturated conditions yielded higher peak stresses in comparison to dry conditions, which is due to the higher densities of saturated samples over their dry counterparts. Fig.3a shows a comparison of peak deviatoric stresses in unreinforced dry and saturated sand fractions. On comparing the results obtained with the results from tests on dry sand fractions [23], the reinforcements in saturated fractions caused only an average 15% increase in the peak deviatoric stress, as shown in Fig.3b and Table 3. On the other hand, addition of reinforcements to dry sand fractions resulted in an average improvement of 58% [23]. The presence of pore water imparts an apparent cohesion to the sand fractions, resulting in agglomeration of sand grains. This decreases the effect of reinforcement on saturated sands. The results can be generalized to state that multi-directional reinforcements are suitable for reinforcing soils in areas where the water table is comparatively low and saturation of soils due to capillary action is negligible.
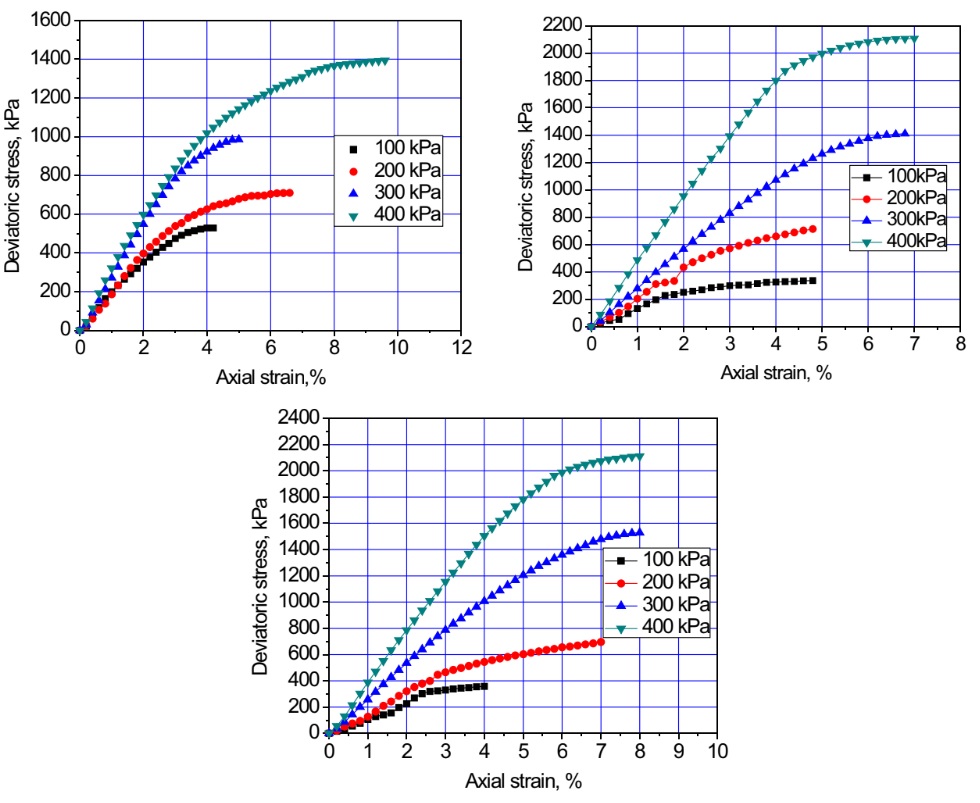
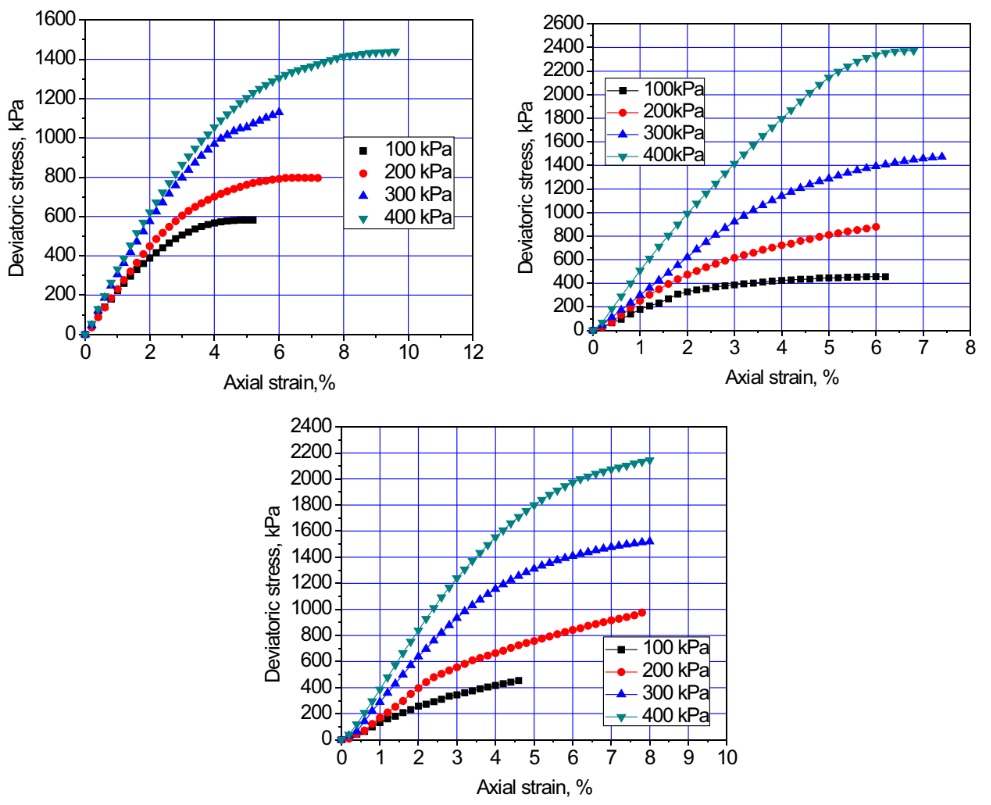
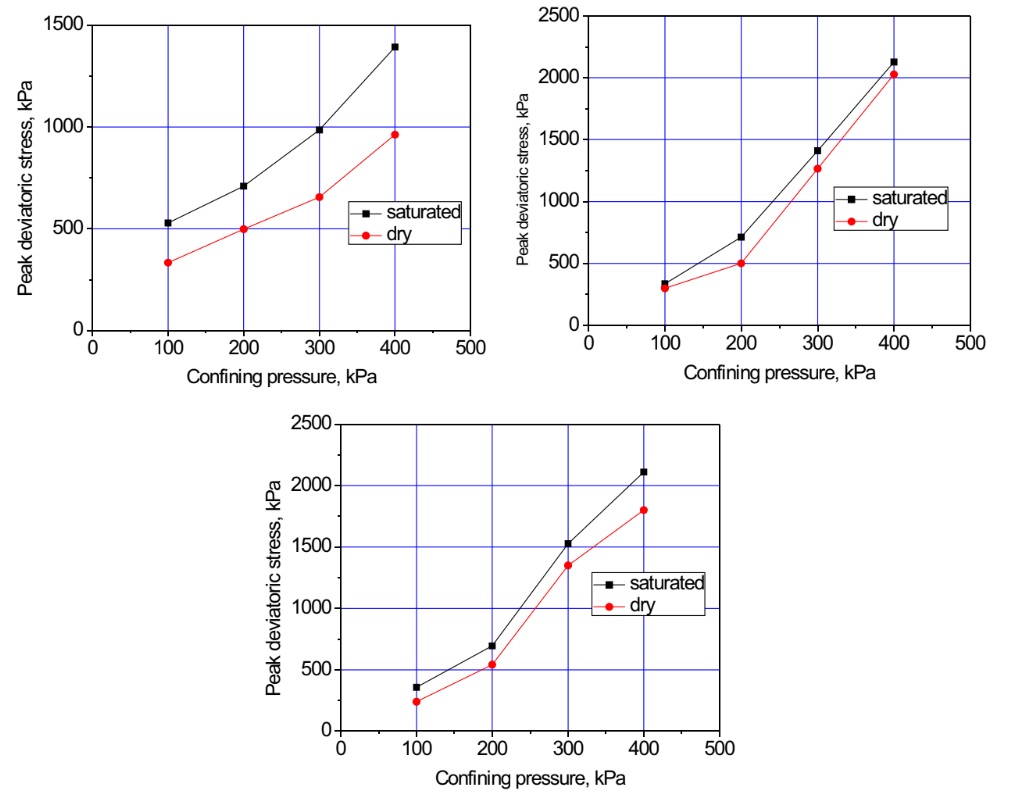
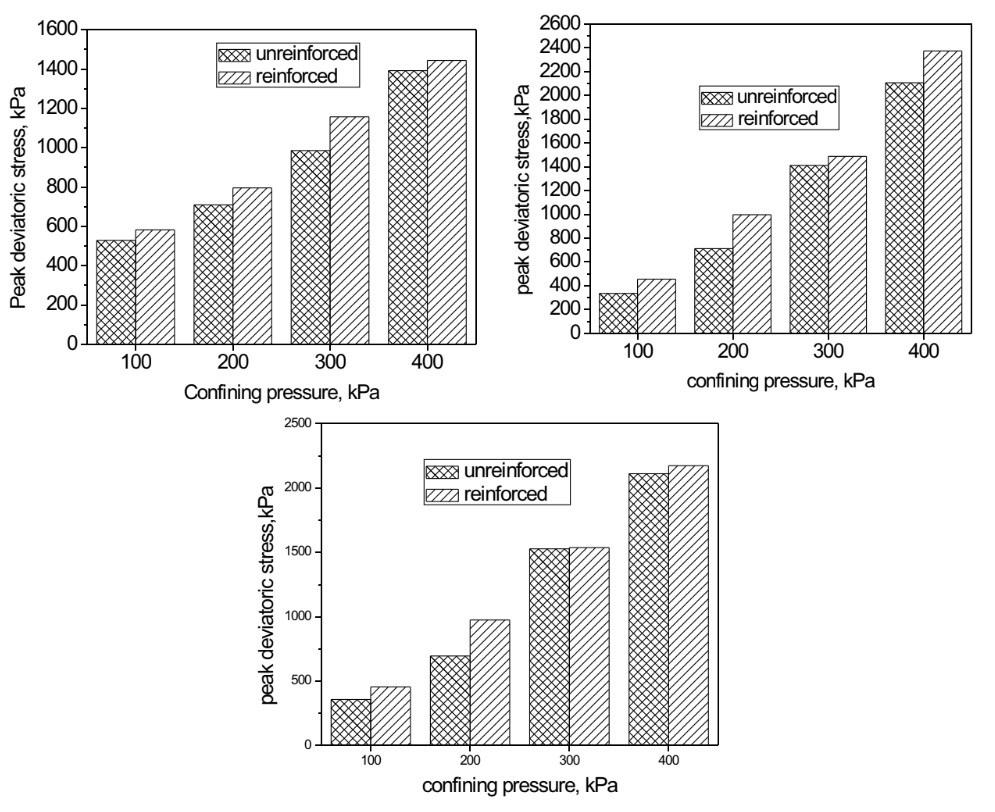
3.1.2 Excess pore pressure-axial strain curves
Plots of excess pore pressure vs. axial strain, in unreinforced and reinforced fractions are shown in Fig.4a-4b. A general dilative behavior is exhibited by medium dense samples, with the result that negative pore pressures were observed. For unreinforced sand fractions, a dilative behaviour was observed throughout. This can be attributed to the relatively higher density of 65%, at which the specimens were prepared. An increase in confining pressure resulted in higher initial and peak pore pressures. In reinforced sand fractions, the samples contracted at lower strains and dilated significantly at larger strains. The pore pressure dissipation improved over their unreinforced counterparts, as shown in Fig.5. The presence of multi directional reinforcements created permeable channels in the soil strata which resulted in higher pore pressure dissipations than unreinforced soil, at the same strains. Further, the presence of plastic reinforcements allows the sample to be compressed to a small extent at lower strains. Soils reinforced with multi-directional reinforcements have been found to possess improved permeability characteristics [22].
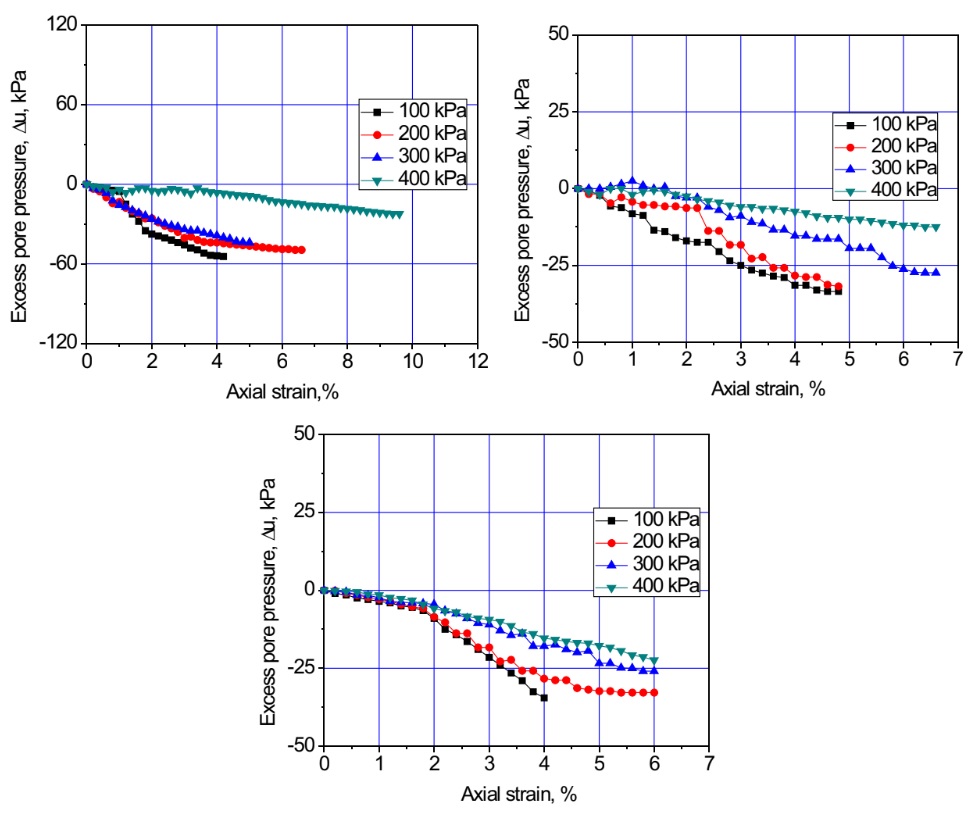
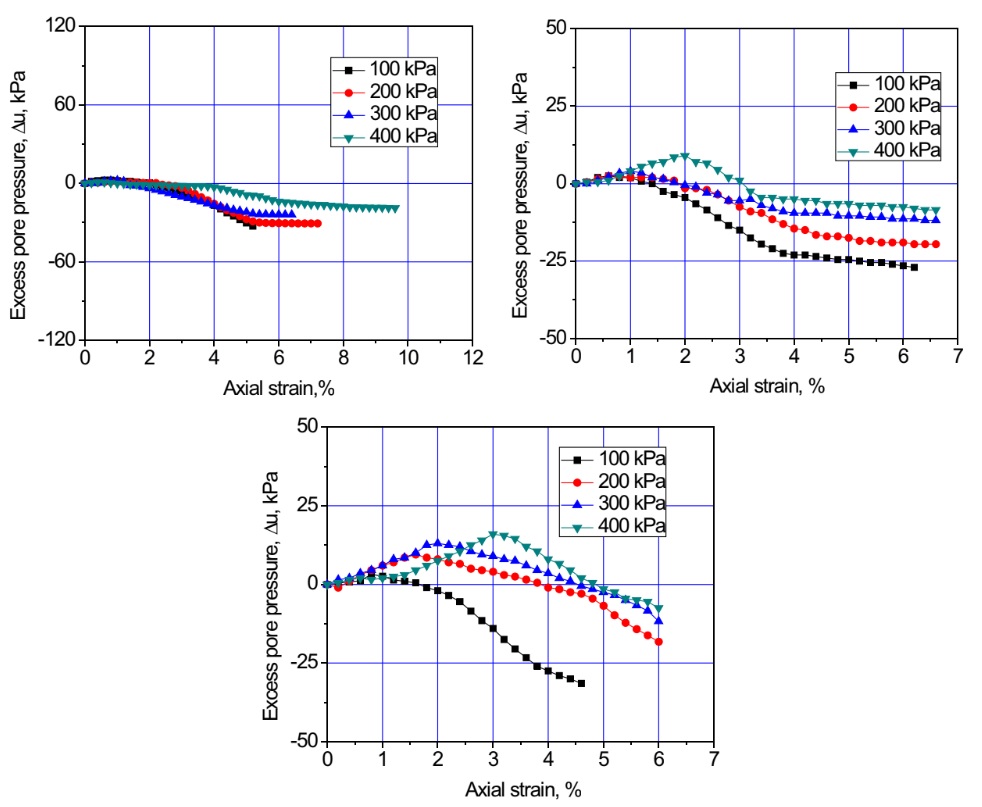
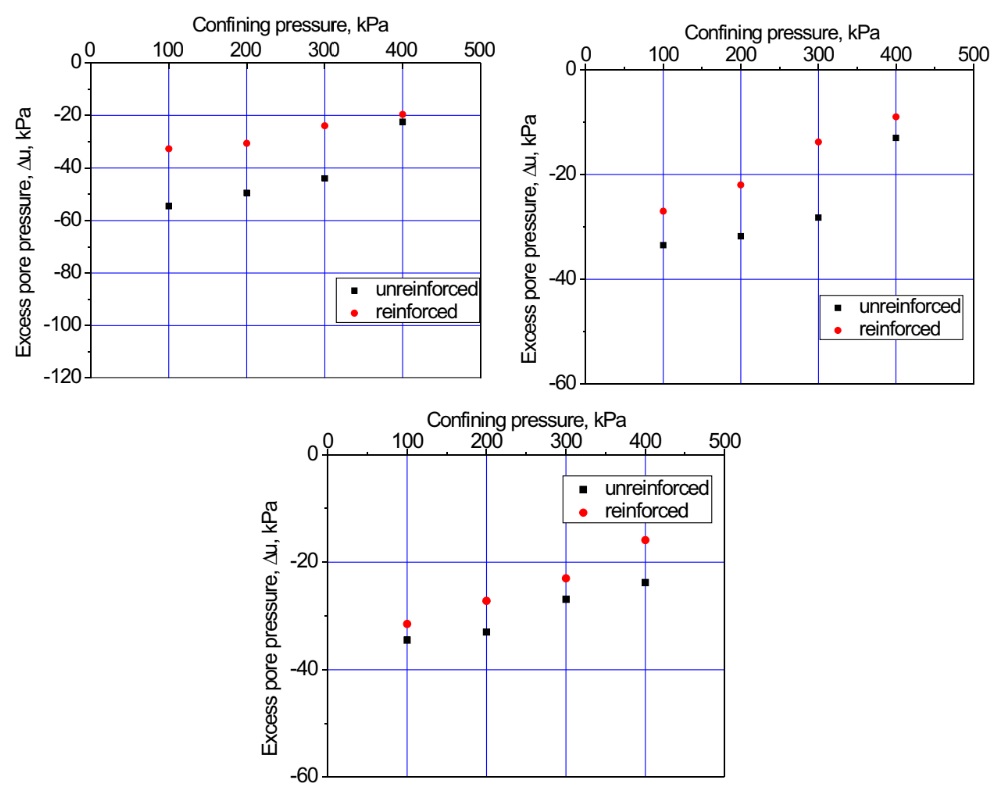
3.2 Consolidated drained tests
Consolidated drained (CD) triaxial compression tests were performed on saturated sand fractions, both in unreinforced and reinforced conditions. In order to ensure that no excess pore pressure is set up during the application of deviator stress, the sample is first consolidated under an equal all-round pressure and subsequently failed by increasing the axial stress under conditions of full drainage. Drained test provides information on volume changes accompanying the application of all-round pressure and the deviator stress and on the stress-strain characteristics of the soil. A burette, attached to the pore pressure lines of the specimen measured the volume change during shear stage.
3.2.1 Deviatoric Stress – axial strain curves
Devatoric stress-axial strain curves were plotted for both unreinforced and reinforced sand fractions, as shown in Fig.6a-6b. It was observed that, generally, the peak deviatoric stress was attained at 4-6% axial strain. Increasing the confining pressure resulted in an increase in peak stresses. For all sand fractions, the amount of strain needed to mobilize full shear resistance in drained tests is generally smaller than that required in undrained tests. This may be attributed to the volume change allowed in drained tests, which allows the samples to take up a comparatively denser degree of packing, even at lower strains. Reinforcing the sand fractions, improved the peak deviatoric stress, although not considerably, as can be seen in Fig.7.
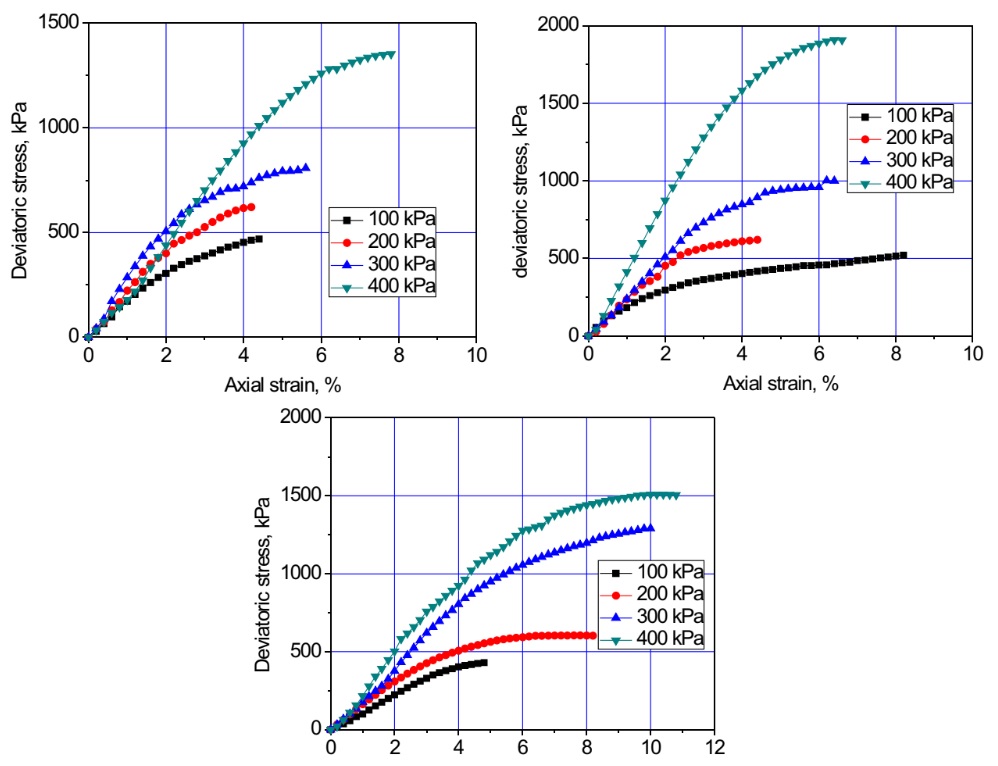
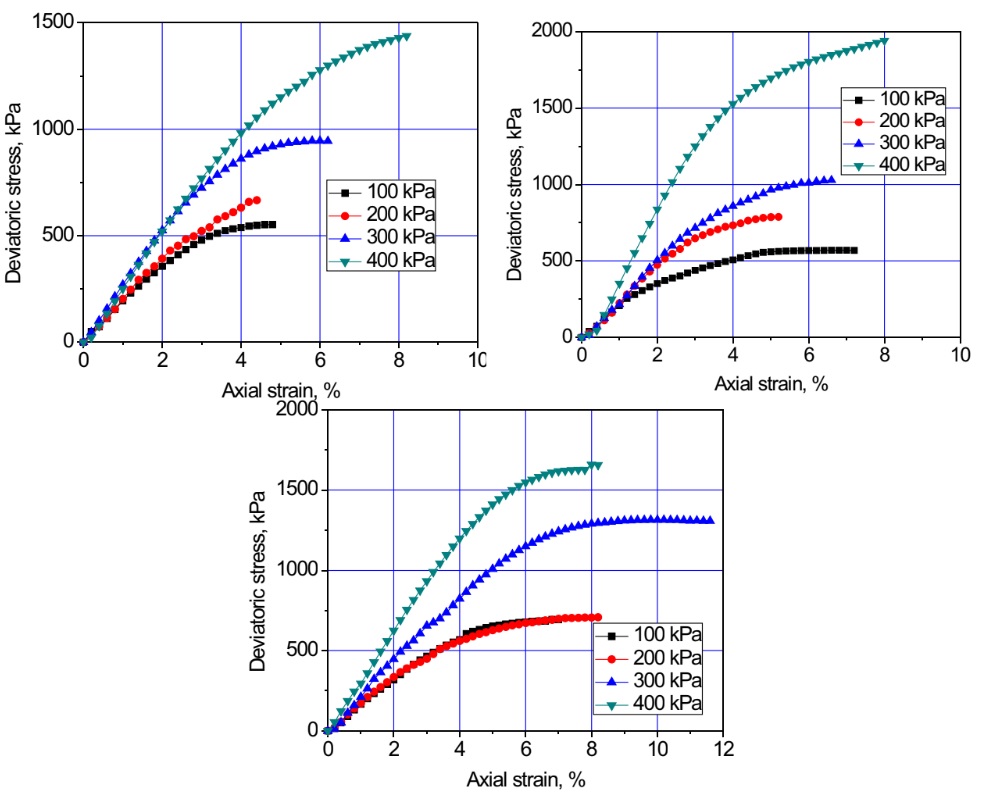
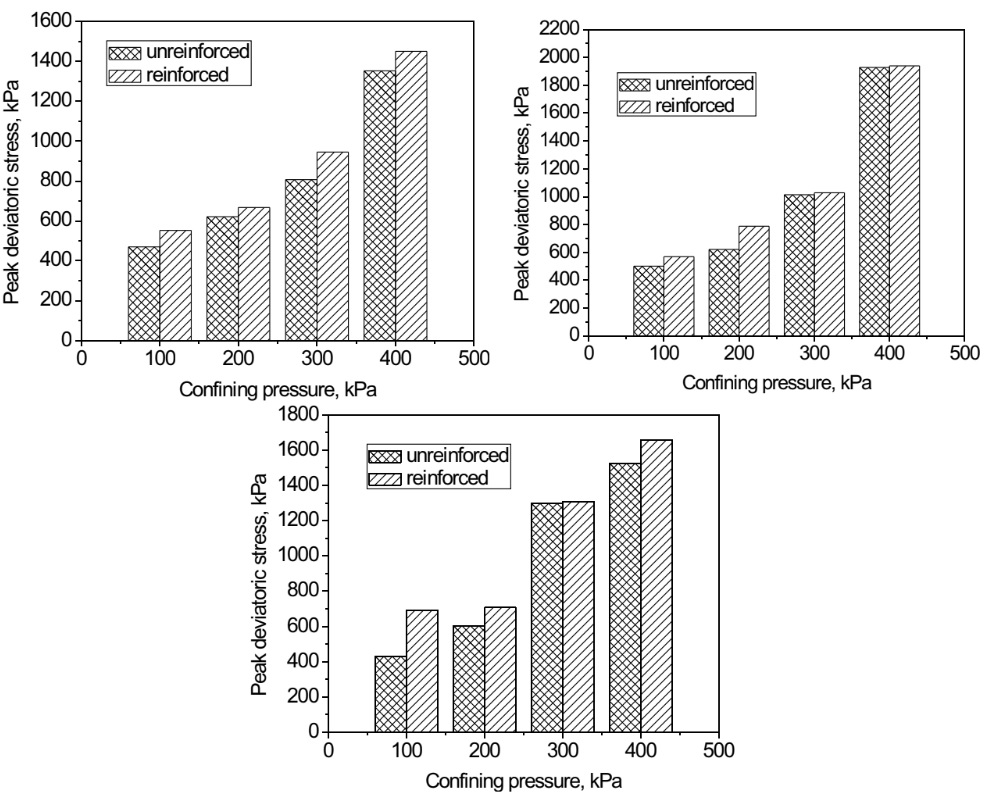
3.2.2 Volumetric strain vs. Axial strain
The responses of volumetric strain to axial strain for saturated unreinforced and reinforced sand fractions are shown in Fig.8a-8b. The behaviour of unreinforced specimen is typical of a medium dense sand specimen. It was noticed that the unreinforced specimens showed a small initial compression at lower strains, followed by a significant dilation up to large strains. This initial compression increased with increasing effective confining stress. On the other hand, with increase in effective confining stress, the amount of dilation decreased, for these specimens. Although similar trends were observed in reinforced specimens, the peak values of volumetric strains were much lesser, as shown in Fig.9. This implies that the presence of multi-directional inclusions prevented the sand samples from undergoing excessive dilation. It was observed that on an average, the peak volumetric strains decreased by about 50% for the reinforced sand fractions. The presence of reinforcing inclusions results in localized confinements in the sand fractions, at the location of placement. This could be the reason behind this significant reduction in peak volumetric strain.
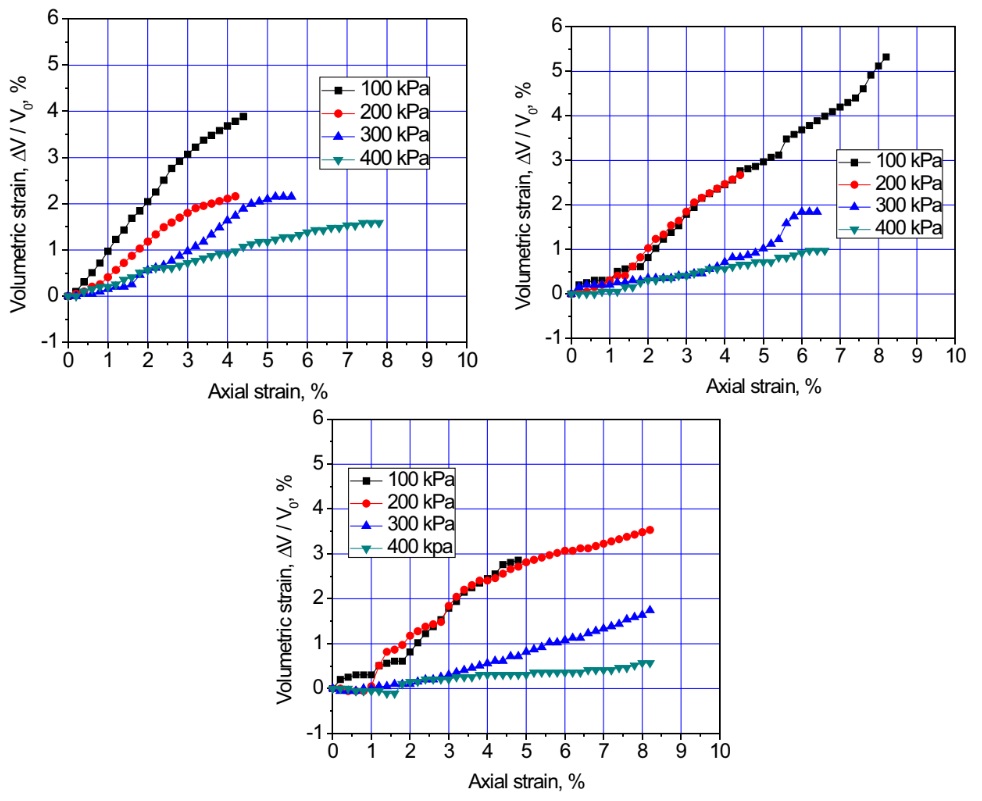
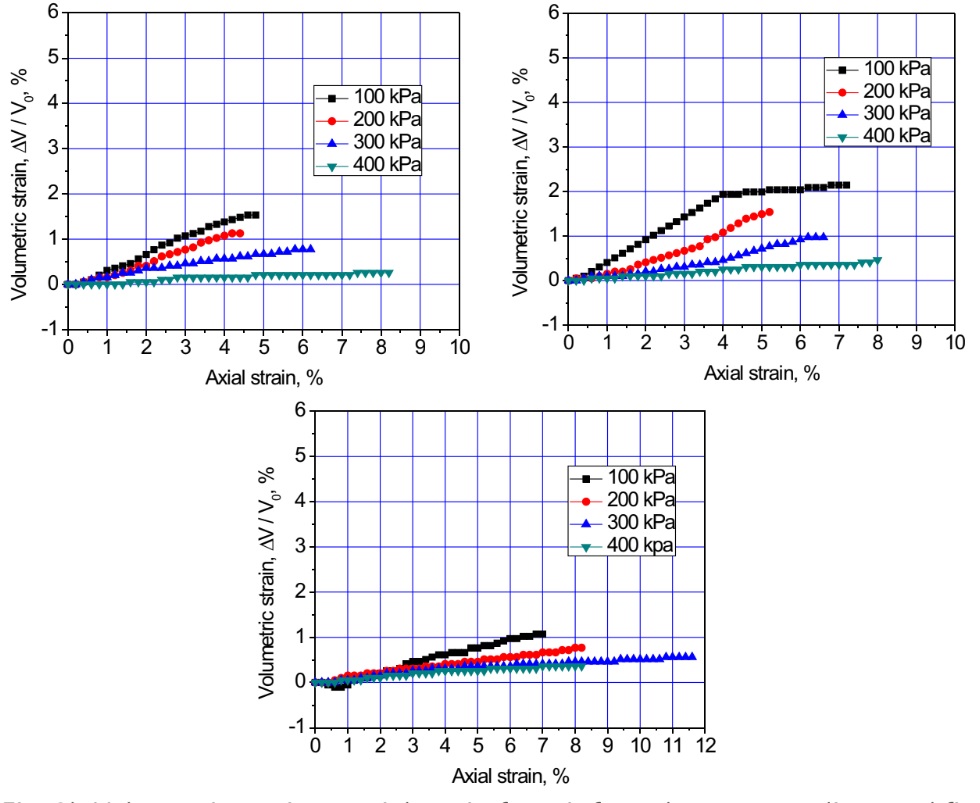
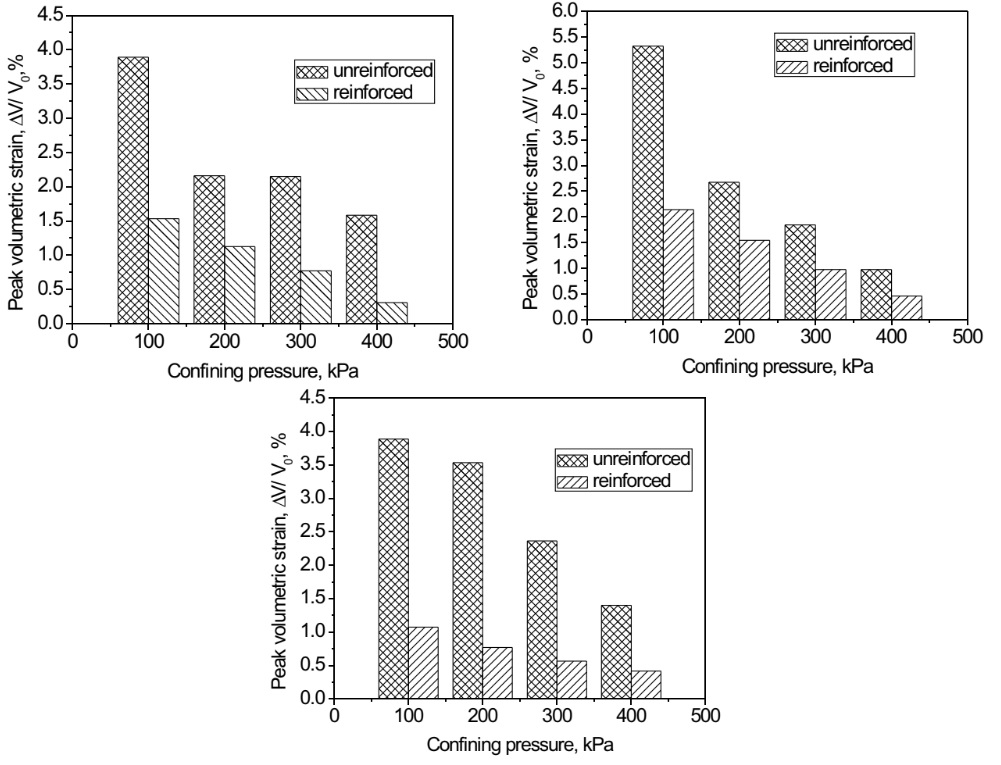
3.2.3 Improvements in strength parameters
Addition of multi-directional reinforcements to saturated sand fractions, improved their response, with respect to the peak deviatoric stress, volumetric strain and the excess pore pressure dissipated. The improvement in peak deviatoric stress was not as high as compared to the improvement in dry sand fractions [Harikumar et al. (2014)]. The peak volumetric strain reduced and the pore pressure dissipation increased significantly. The improvements in these parameters are quantified in Table 3.
Table 3. Improvements in strength parameters for reinforced soil fractions under saturated conditions
Sand | Improvements in soil parameters, % | ||
Peak deviatoric stress | Volumetric strain | Excess pore pressure | |
Coarse | 10 | -50 | 13 |
Medium | 22 | -75 | 31 |
Fine | 17 | -60 | 33 |
Although triaxial tests are small scale tests to directly extend the results of the study to a larger scale field prototype, the results of a few of the field studies conducted by researchers, with 3D reinforcements seem to be in agreement with the findings in this paper [21,22]. Hence extensive investigation is needed to determine the effect of overall size and shape of the reinforcement, reinforcement material properties and its economy before actually applying them in the field.
CONCLUSIONS
In the present study, an attempt has been made to study the reinforcing effects of plastic multi-oriented reinforcements on the response of saturated sand fractions.
A series of drained and undrained triaxial compression tests were performed on 50 mm diameter saturated samples, both in unreinforced and reinforced conditions. The effect of grain size, confining pressure and the presence of reinforcing inclusions on the excess pore pressure dissipated and the volumetric strains were investigated. The following conclusions were notable:
a. When the confining pressure was increased from 100 to 400 kPa, both reinforced and unreinforced sand fractions showed improvements in peak deviatoric stresses.
b. The peak deviatoric stresses obtained CU tests on saturated sand fractions were much higher, compared to those on dry sand fractions, presumably due to the higher densities of saturated samples.
c. The amount of strain needed to mobilize full shear resistance in drained tests was found to be smaller than that required in undrained tests, for all sand fractions in a medium dense state, which may be due to the volume change that is allowed in drained tests.
d. Reinforced samples in undrained tests showed higher pore pressure dissipation and significantly lesser volume changes than their unreinforced counterparts.
e. Peak deviatoric stresses for reinforced sand fractions in saturated conditions were found to be slightly higher than their unreinfored counterparts, which means that reinforcements were not much effective in saturated conditions, as they proved under dry conditions [23].
About author
The author is an Assistant Professor (Civil) at the College of Engineering, Vadakara, Kerala (Under Govt. of Kerala). He specializes in Fluid mechanics, Open channel hydraulics, Geotechnical Engineering and Engineering Geology He is a Gold medalist in M.Tech Environmental Geotechnology, NIT Calicut and recipient of the Excellent Performance Award 2004-2005, Civil Engg. Department, CET. He has written several journals and has cited his papers across different national and international conferences.
REFERENCES
- Wasti.Y and Butun, 1996, Behaviour of model footings on sand reinforced with discrete inclusions.
- Fleming, I.R., Sharma, J.S., Jogi, M.B., 2006. Shear strength of geomembrane–soil interface under unsaturated conditions.
- Iizuka, A., Kawai, K., Kim, E.R., Hirata, M.. Modeling of the confining effect due to the geosynthetic wrapping of compacted soil specimens.
- Katarzyna, Z.A. Shear strength parameters of compacted fly ash–HDPE geomembrane interfaces.
- Latha, M.G., Murthy, V.S Investigations on sand reinforced with different geosynthetics.
- Park, T., Tan, S.A. Enhanced performance of reinforced soil walls by the inclusion of short fiber.
- Patra, C.R., Das, B.M., Atalar, C. Bearing capacity of embedded strip foundation on geogrid-reinforced sand.
- Varuso, R.J., Grieshaber, J.B., Nataraj, M.S. Geosynthetic reinforced levee test section on soft normally consolidated clays.
- Yang, G., Wang, Y. Strength property of RC net reinforced earth retaining structure and its experimental study.
- Yetimoglu, T., Inanir, M., Inanir, O.E. A study on bearing capacity of randomly distributed fiber-reinforced sand fills overlying soft clay.
- Chandrasekaran, B., Broms, B. B. & Wong, K. S. Strength of fabric reinforced sand under axisymmetric loading.
- Haeri, S.M., Nourzad, R., Oskrouch, A.M. Effect of geotextile reinforcement on the mechanical behavior of sands.
- Venkatappa Rao, G., Dutta, R.K., Ujwala, D. Strength characteristics of sand reinforced with coir fibers and coir geotextiles.
- Gray, D.H., Ohashi, H. Mechanics of fiber reinforcement in sand.
- Gray, D. H., and AI-Refeai, T. “Behavior of fabric-versus fiber-reinforced sand.”
- Al-Refeai, T. Behavior of granular soils reinforced with discrete randomly oriented inclusions.
- Gopal Ranjan, Vasan, R.M., Charan, H.D. Behavior of plastic fiber-reinforced sand.
- Kaniraj, S.R., Gayathri, V. Geotechnical behavior of fly ash mixed with randomly oriented fiber inclusions.
- Yetimoglu, T., Salbas, O. A study on shear strength of sands reinforced with randomly distributed discrete fibers.
- Park, T., Tan, S.A. Enhanced performance of reinforced soil walls by the inclusion of short fiber.
- Zhang M.X., Zhou H., Javadi A.A, Wang Z.W. Experimental and Theoretical Investigation of Strength of Soil Reinforced with Multi-Layer Horizontal Vertical Orthogonal elements.
- Lawton E.C, Khire M.V, Fox N.S. Reinforcement of Soils by Multioriented Geosynthetic Inclusions, Journal of Geotechnical Engineering 119 (2).
- M Harikumar, N Sankar, S Chandrakaran. A regression model for predicting the response of sand reinforced with random multi-oriented reinforcements, EJGE, Vol. 19 [2014], Bund. Q
- Bishop, A.W., Henkel, D.J. The Measurement of Soil Properties in the Triaxial Test. William Clowes and Sons Ltd., London and Beccles
- Head, K.H. Manual of Soil Laboratory Testing, vol. 2. Pentech Press, London, UK.
- M Harikumar, N Sankar, S Chandrakaran, An Alternate An Alternate Method of Saturating Sand Specimens in Triaxial Tests, IJERT, Vol 3, Issue 9.