Bridge management plays a significant role in the sustainability of infrastructure. This has become more relevant since the world over bridges are aging and most countries have a population of aged bridges. Sustainability and maintenance within the Bridge Management System (BMS) support the principles that optimize the environmental and economic benefits of bridge structures. This optimization is achieved by activities that include maintenance, rehabilitation, restoration, and replacement to maximize the life cycles of any bridge structure. Sustainability is ensured by a reduction in costs and reduced raw material usage which impacts the environment due to construction to the minimum thereby preserving the environment for the future generation. In today’s world sustainability is the most discussed topic in the engineering community. The sustainable design and maintenance of infrastructures using Life-Cycle Cost Analysis are extensively used. This results in cost-effective solutions to ensure that desired level of performance is restored. Cost estimates for such interventions and the resultant enhancement in service life contribute to an important index in life cycle analysis. The purpose of a Life-Cycle Cost Analysis [LCCA] is to estimate the overall costs of project alternatives and to select the design that ensures the facility will provide the lowest cost of ownership consistent with its quality and function. Such LCCA is more realistic if the intangible benefits resulting from Social, Economic, and Environmental parameters are also accounted for. It is important to consider the life cycle cost for users from the social point of view. UBMS Analytics enables such LCCA evaluation due to the Socio-Economic parameter, data for which is available within the system for every bridge.
- INTRODUCTION:
The sustainability of bridge infrastructure encompasses the need to retain the present environmental, social, and economic conditions and also ensure they are retained for future generations. Rampart growth to maintain development should be managed to ensure sustainability. The sustainable bridge infrastructure should support the basic principle of managing economic, social, and environmental balance through effective management of bridge infrastructure. [7,8] Bridge infrastructures can be considered to be sustainable development if cost-effectiveness is ensured within the given amount of material and energy resources they consume during their construction, maintenance, and rehabilitation life cycle phases. This can only be achieved through developing LCCA that addresses sustainability issues at the planning and designing, construction, operation, and maintenance stages. [4]
It is very important to monitor the bridge development for realistic and economic costs from designing, constructing, maintaining, and rehabilitation of bridges. This process could result in the sustainability of bridges during infrastructure growth. It should also result in reducing the total cost of bridges during life cycle management in the future. Sustainable bridge constructions refer to the minimized emissions of harmful products during bridge construction. [8] It is equally important, to design durable bridges with minimum serviceability of around 100 years as stipulated in the recent design standards.
The challenge to maintaining sustainability and economic growth in parallel arises from the rapid pace of enhancement of networks. To ensure economic development, infrastructures are created. It is essential to adopt the strategies which will enable us not only to build new bridges sustainably but also to develop methods to extend the service life-cycle of existing structures sustainably. Strategies need to be developed for keeping existing bridge infrastructures sustainable and building new sustainable bridge infrastructures.
A Unified Bridge Management System [UBMS] provides a possible solution by ensuring the Sustainability of bridge infrastructure is kept in focus in all the Analytics tools it deploys. Structural health monitoring integration with UBMS offers realistic observations not linked to any bias or judgment. In this, one has developed a procedure to modify the Cause matrix whenever the Performance is observed to have decreased. This ensures that Performance monitoring based on SHM is linked to the decision-making process within UBMS. This combined with the resultant cause matrix offers reliability in the estimation of the deterioration model and further in the risk analysis and optimization of funds. [5] To ensure optimization occurs after detailed financial due diligence, UBMS deploys Life Cycle Cost Analysis [LCCA]. LCCA is one such tool within UBMS Analytics that remains focused on sustainability.
LCCA for a bridge is the present value of total costs of the bridge that appear during the entire life span which includes initial investments, maintenance, rehabilitation, and repair costs in the bridge. LCCA can be bifurcated into two Costs and Benefits namely [1,4,13]
- Direct costs and benefits
- Indirect costs and benefits.
These are further sub-categorized into initial, operation, repair, and maintenance costs and direct and intangible indirect benefits. Research has been conducted on the evaluation of the economic impacts associated with the service life of the bridge and the resultant LCCA is more comprehensive.
- Linking Life Cycle calculations in Analytics of UBMS
LCCA of bridge infrastructures is project-based, which was used for determining the detailed analysis. The importance of the benefit-cost ratio in reducing the various travel-related costs such as – vehicle operating costs, maintenance costs, and environmental impact costs has to be considered. Vehicle Operating Costs [VOC] is essential for users because it minimizes operating expenses when bridge infrastructure is enhanced and offers improved operational benefits. Primarily the significance of Value of Time [VOT] is critical for both passengers and freight shipment. These benefits that arise from VOC and VOT savings are tangible and considered in normal LCCA.[2] This is a general practice adopted in the evaluation of the Benefit-Cost ratio for any infrastructure including bridges. Apart from these direct costs and benefits, there are few intangible impacts due to the existence of the bridge. A few of them are the negative impact the bridge has on the surrounding environment /forest or greenery due to increased pollution and gas emissions. The positive impacts are the growth in the economy of the areas connected by the bridge, the increase in connectivity the bridge offers, and the impact on the social life of the populations that use the bridge by offering more opportunities for employment due to ease in travel. [7,9]
Incorporating such intangible benefits into LCCA is a challenge. The estimation of all such intangible benefits and costs is dynamic and varies with a period. Unless observations and records are available within bridge management to link the dynamics of such changes, it is difficult to estimate and account for such costs and benefits. Normal LCCA evaluations neglect such inclusions. Within UBMS, the existence of the Socio-Economic parameter records the changed scenario on the social and economic aspects due to the bridge. Such records are updated every time an inventory level inspection is carried out. Linking the Socio-Economic parameter to evaluate the intangible costs and benefits was overcome by records and technical review of various previous studies. [1,6,7,8]
UBMS applies for such intangible costs and benefits within LCCA calculations and the resultant Benefit-cost ratios are found to be more dynamic and realistic. The benefits are enhanced as the savings are more practically enhanced if the intangible benefits for bridge infrastructure accrued from socio-economic aspects, which accounts for all the benefits arising indirectly due to social, economic, and environmental are considered in the financial calculations. Bridge infrastructure results in economic benefits arising from the transportation of people and goods over the network. The accessibility, safety, and movement on a bridge are dependent on the population which uses the bridge. This increases the social importance of the bridge. LCCA of such bridge structures must be based on dynamic, robust, and real-time information. Within UBMS, maintenance and rehabilitation decisions have to be made based on correct factual data to ensure that the bridges are managed to ensure that the designed performance over the entire life span is ensured. The approach professed by all existing Bridge management relies at best on observation of symptoms and identification of the cause of distress. This approach creates a bias based on the judgment of the engineer inspecting the bridge. As engineers, one needs to rely more on factual data. Records of performance monitored periodically under live load are one such factual data series for the bridge. Performance of aging bridge structure under live loading can be monitored. This performance data will need to be used for triggering required maintenance interventions. UBMS applies such data, wherein the deterioration model is twitted dynamically based on real-time monitoring data of the bridge under live load conditions. Such monitoring will need to be periodic and can be for short time durations. Such periodic monitoring results in time series data revealing the possible decrements in performance. Such decrements in performance are linked by our algorithm to increments in distress. Deterioration models created based on such evaluated increments in distress results in more realistic prediction about the future behaviour of the bridge. When engineering decision-making is based on reliable and factual data, it is natural to have equally reliable and factual data for LCCA calculations. Linking the Socio-Economic parameters within UBMS to LCCA ensures this is adhered to.
- LIFE CYCLE COST ANALYSIS [LCCA]
LCCA is a cost-centric approach for determining the most cost-effective alternative. The lifespan of various bridge components is determined by their rate of deterioration. The pier and foundation are more vulnerable to deterioration because of environmental or collision impacts. The environmental exposure of steel components influences how quickly the steel corrodes. A bridge is a long-term, multi-year investment, and the cost to the owners for a bridge is never a one-time expense. Bridge components require preservation and maintenance actions to counter the effects of deterioration throughout their Service Life. Few Bridge components like bearings and expansion joints may even require replacement as their Service Life is less than that of the bridge. [1,6,7,8,14] LCCA may also be used to evaluate competing design alternatives. In such cases, the bridge’s service life is used as the analysis period. Each design alternative must first show it reliably achieves project requirements such as service life, structural stability, and desired level of maintenance. [10,17]
LCCA is done assuming standard routine maintenance and periodic rehabilitation interventions every few years. Annual routine maintenance is essentially required. Periodic rehabilitation intervention can be triggered by the observation of reductions in performance. EN 1504[18] has defined 3 primary processes (Mechanical, Physical, and Chemical) as primary processes causing deterioration in bridge elements. Estimation of such deterioration process is evaluated. Based on such estimation, plans for rehabilitation intervention to be provided are put in place. Cost estimates for such rehabilitation intervention are also calculated. Such intervention results in enhancement in life and performance in bridge elements. This is evaluated and can be adopted in LCCA. The changes in Internal Rate of Return [IRR] calculations will be evident. This can be one of the important decision-making process parameters. Furthermore, additional decision-making parameters need to be introduced into the decision-making process to ensure realistic and cost-effective solutions. Additional parameters are outlined in the Handbook for Implementing IBMS/UBMS.[16] One needs to evaluate if any of the life spans are compromised. Three life spans are Physical life span, Functional life span, and Economical life span. Physical life is compromised when its integrity is compromised. Functional life is compromised when any one of the four Functional parameters has ratings lower than 5. Economic life is compromised when the cost associated with the utilization is negative. The benefits are lower than the costs required to keep the bridge functioning. With LCCA used as an Analytics tool, one can introduce all such additional parameters in the decision-making process.
LCCA is initially prepared based on standard annual maintenance and planned rehabilitation intervention every 7th year after the initial 15-to-20-year lifetime of the bridge. Such an LCCA results in the evaluation of IRR for the entire life of the bridge. When the performance monitoring system is put in place, the deterioration model is generated. Based on the deterioration model created, one can define the type and volume of rehabilitation intervention and the cost involved. This cost estimate and which year the rehabilitation intervention will be introduced are incorporated into the LCCA sheet. Enhancements in service life spans are accounted for in calculations of IRR. Changes in IRR will be observed. Increments in IRR will be taken as a trigger to put in place the planned rehabilitation intervention. Decreased IRR will essentially mean a closer look at the proposed rehabilitation intervention and the need to redesign the rehabilitation interventions. One can also evoke a more comprehensive approach to the decision-making process by including other critical parameters of the decision-making process.
Terminologies in LCCA [1,2,4,8]
1. Time Value of Money: The value of money today and money that will be spent in the future are not equal. This concept is referred to as the “time value of money.”
2. Discount Rate: The exponent value is used to compute the equivalent present value of a future cost; the effective discount rate accounts for inflation, the relative financial risk of an investment, and the time value of money; compare the interest rate, inflation rate, and real discount rate.

Where i= Real Discount Rate
3. Present Value: The value of a cost incurred at a future time is expressed as the amount that would be equivalent if that cost is incurred now, computed as a function of the discount rate and period between and the anticipated time when the cost will be incurred.
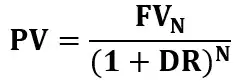
Where: PV = Present Value; FV=Future Value at Year N; DR=Discount Rate
4. Benefit-Cost Ratio: The benefit-cost ratio (BCR) is a ratio used in a cost-benefit analysis to summarize the overall relationship between the relative costs and benefits of a proposed project. BCR can be expressed in monetary or qualitative terms. If a project has a BCR greater than 1.0, the project is expected to deliver a positive net present value to an Owner and its investors.

Internal Rate of Return (IRR)
The internal rate of return is the interest rate received for an investment consisting of payments (negative values) and income (positive values) that occur at regular periods. It is expressed as a percentage per annum. IRR is closely related to NPV. The discount rate is such that the net present value of a stream of present and discounted future costs and savings or revenues is exactly zero. However, IRR always does not give a mathematical solution and is not a stand-alone economic assessment in a whole life cycle cost analysis.
Data needed to establish the input parameters for the LCCA: [3,4]
All the costs and parameters considered here are highly uncertain. Therefore, each term is discussed here in detail as to how the probabilistic nature of each parameter can be included in the analysis.
- Capital Cost: In a bridge rehabilitation project, capital cost will be the initial maintenance cost. It will include the material cost, labor cost, user delay costs, etc.
- Maintenance, Monitoring, and repair cost: In estimating the maintenance cost the updated failure probability in each maintenance stage needs to be known. If there are data available for the failure of each repair strategy, it is possible to evaluate the probability related to that effect.
- Rehabilitation Cost: The costs associated with the rehabilitation of bridge elements should be estimated for different types of elements and the different rehabilitation alternatives applicable for each element type. Together, elements and alternatives give estimates of the costs for element rehabilitation corresponding to different rehabilitation alternatives.
- Routine Maintenance Costs: The costs associated with the routine maintenance of bridge elements can be estimated in the following ways. First, these costs can be developed as a function of the material type, condition, location, average daily traffic (ADT), highway classification, and other key factors for each bridge element. Second, these costs can also be estimated by first estimating the quantity of different routine maintenance activities performed on a type of element per year as a function of element condition, material type, ADT, highway classification, environment, and other factors.
- Failure Cost: The expected cost of failure needs to be considered in the life cycle cost of structure to decide about a cost-effective solution with the risk of failure included. Due to uncertainties associated with structural properties, loads, and environmental conditions the cost of failure is a random variable.
The following steps define the LCCA Methodology [1,4,6,8,11,13,]
- Identification of Alternatives Design: The life cycle cost analysis (LCCA) process identifies reasonable design alternatives and a preservation strategy for bridges. For each proposed alternative, identify initial construction activities, the necessary future maintenance activities, and the timing of those activities. At least two mutually exclusive options must be considered and the economic difference between them is assumed to be attributable to the cost of each. There are different alternatives for bridge maintenance and improvement for a given set of distress characteristics. Each alternative is then analysed with the alternative’s activity profiles and cash flows for project-level decisions.
- Determine activity timing: A schedule of activities for each project alternative is created using this information. After identifying the component activities for each competing project alternative, maintenance plans for each alternative are developed. This strategy effectively results in a schedule of when future maintenance activities will take place when funds will be spent, and when and for how long the work zones are established.
- Estimation of costs: Following that, activity costs are estimated in detail. Best practice in LCCA requires direct costs/ expenditures (such as construction, maintenance, rehabilitation, or replacement activities) to be included. LCCA does not require the calculation of all expenses involved with each alternative. Only costs that show the differences between alternatives should be investigated.
- Estimate user costs: In LCCA, user costs of primary interest include vehicle operating costs, travel time costs, and crash costs. User costs are primarily attributable to the functional deficiency of a bridge such as a load posting, clearance restriction, and closure. These functional deficiencies may cause higher vehicle-operating costs because of detours, lost travel time, and accidents.
- Determine Life Cycle Costing (LCC): Once the expenditure streams for the various competing alternatives have been determined, the goal is to calculate the total LCCs for each alternative.
Typical LCCA in UBMS consists of the following headings as given below:
The sheet will have all calculations compiled for successive Years which are maintained in the first column. The next few columns are for FINANCIAL COST which arises from Capital Investments, Regular Maintenance Costs, Periodic Rehabilitation Costs, and the Total of all costs which are then converted to Economic costs. The next columns are for DIRECT BENEFITS which arise due to VOT [ Vehicle operating time-saving benefit] and VOC [ Vehicle operation cost saving] Total direct benefits are in the next column.
Finally, the next few columns are for INDIRECT BENEFITS which comprise Benefits due to Social Impact, benefits due to Economic growth, benefit due to saving of time due to non-use of Alternate route as the bridge is functional, and finally the costs due to Environmental impact of the bridge on the surrounding area. The total of all Indirect benefits is calculated in the next column.
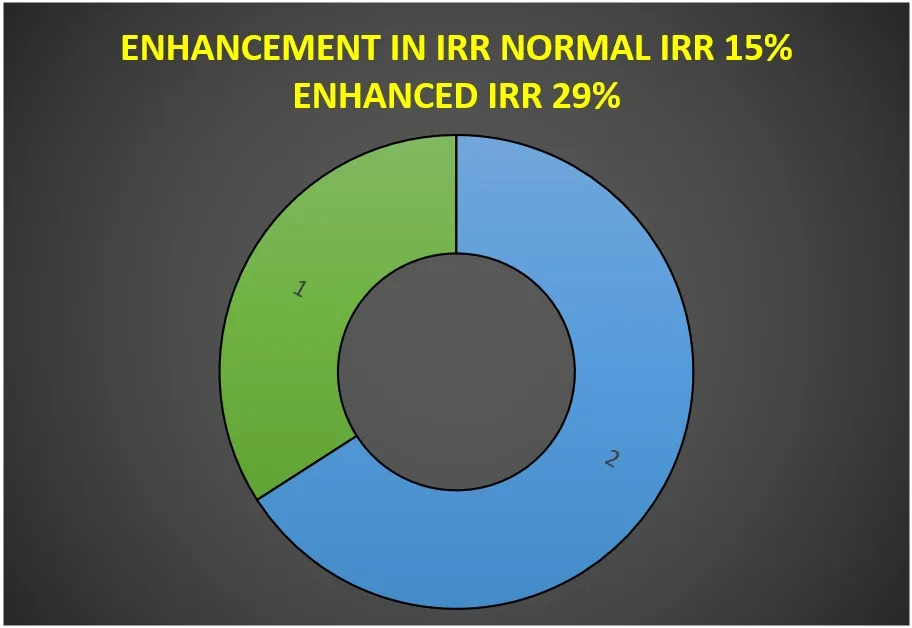
The next column is the summation of Direct and Indirect benefits and then the actual evaluation of the Benefit-Cost ratio for that year. This LCCA within UBMS results in an Enhanced and realistic Benefit-Cost ratio [BCR] which is greater than the conventional BCR by nearly double or more depending on the intangible benefits accrued due to Indirect Benefits that accrue due to the bridge structure. Such application of LCCA results in enhancement in IRR evaluation to the extent of over 80%. A typical example is shown below.
RELEVANCE OF SUSTAINABILITY
Using Bridge Management System enables maintenance, strengthening, repair, and rehabilitation of bridges if interventions are applied timely. This ensures maximizing the life span of bridges without the need for replacement. Such interventions ensure to reduce the cost of the life cycle and reduce the impact on the environment by reducing the usage of raw materials. These interventions enhance the environmental and economic management in the entire life cycle by maximizing the benefits for the given costs. The timely provision of interventions ensures economic benefits by managing the cost-effectiveness in the design of such interventions, by ensuring to reduce the detour travel time, congestions, and traffic jams delay resulting in avoidance of productivity loss and its impact on the reduction of GDP of the area of influence of the bridge. Such intervention also ensures enhanced social benefits by reduction or avoidance of failure/ collapse and increased reliability of network which impacts the safety during travel, life loss, timely delivery of goods and raw material which are crucial to maintaining the productivity which ensures stable GDP. Environmental goals of minimizing the carbon emissions are ensured by avoidance of traffic jams, and longer journey time due to detours in case of closure of the bridge for a longer period when collapse occurs.
Sustainability ensures to delivery of the optimal level of service safely to existing and future bridge users in the most cost-effective way, LCCA enables the owners to anticipate, and plan investments in a very cost-efficient manner. LCCA also enables us to prioritize investments on the assets that need it most. LCCA also enables optimization of the costs essential to ensure the bridge is functional for the entire service life span. This optimization is ensured by a risk-based analysis to identify cost-effective investments during conceptualization, designing, construction, and during the entire operational life of the bridge.
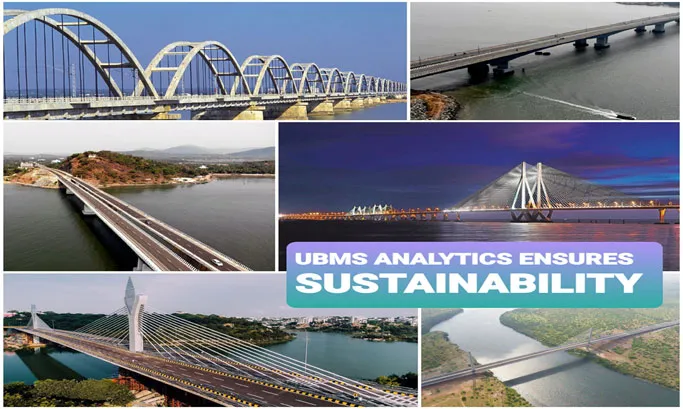
The sustainability objectives are as follows: [1,3,6,8,10,12]
Reduce virgin material use; Optimize waste stream; Reduce energy use; Reduce emissions to air; Maintain or improve hydrologic regime characteristics; Maintain biodiversity; Engage community values and sense of place; Improve safety; Improve access and mobility; Improve local economy; Increase lifecycle efficiency; Promote innovation.
For example, the following sustainability practices are related to the planning stage of a bridge:
Addressing scour; Bridge aesthetics; Importance of safety for bridge users; Maintain or improve access for bridge users including pedestrians and cyclists; Maintain or improve access for transit; Maintain or improve aquatic ecosystems; Embrace public participation; Reduce bridge greenhouse gas emissions; Maintain or improve terrestrial ecosystems; Resilience; Durability; Reduce noise pollution.
The final goals of sustainability are ensured by proper evaluation and implementation as derived from Bridge LCCA. Reduced cost of construction by increased use of local raw materials, reuse of material, and ensuring recycling of waste generated during the life span of the bridge are a few examples of efficient LCCA management. Such practices result in less consumption of fuels during the entire life span of the bridge from construction to decommissioning of the bridge. The sustainability analysis provides direction for improving the sustainability of bridge infrastructure projects and the rationale for undertaking specific actions. “Sustainable development is a development that meets the needs of the present without compromising the ability of future generations to meet their own needs.” In the bridge engineering community, sustainability means planning, designing, constructing, and managing bridges that maintain a balance between the three pillars of sustainability: social, economic, and environmental considerations. While there are many definitions for sustainability, which means supporting the natural, social, and economic systems upon which One depends now and will depend in the future. A bridge constitutes a large investment of natural, material, financial, and human capital and thus has the potential for significant positive and negative effects on the environment and society throughout its long life. The charts indicate cost distribution (fig:1) and benefits(fig:2), in which 34% show Capital Cost, 37% show regular Maintenance and 29% show periodic maintenance, 34% depict net benefits, and 66% show cost-benefit.
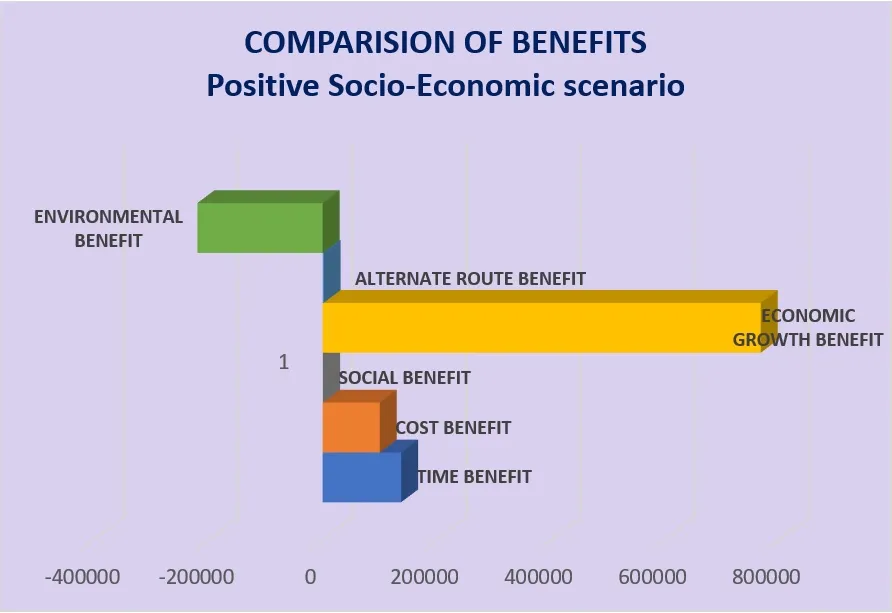
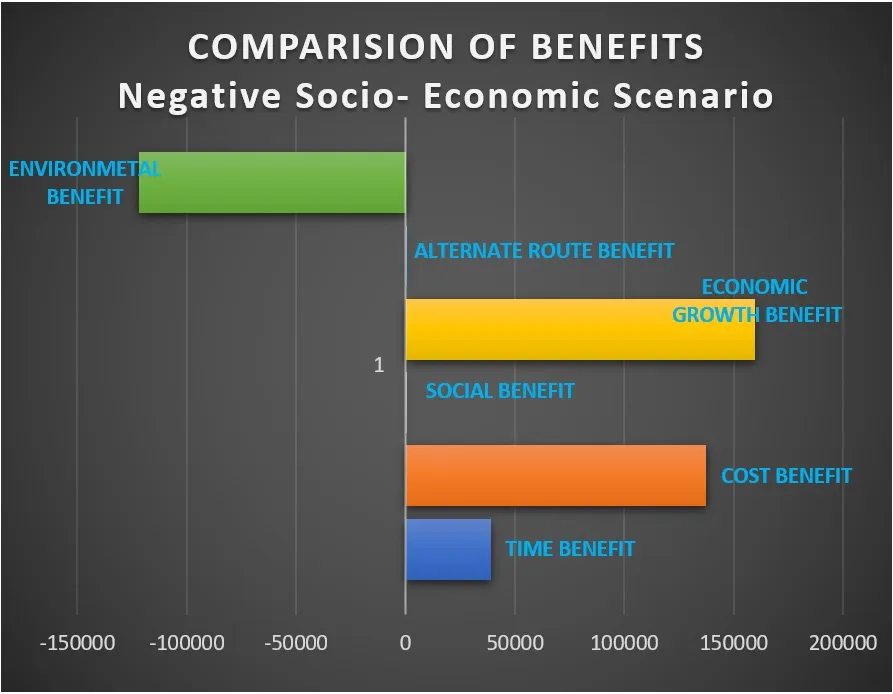
LCCA’s IMPACT ON RANKING PROCESS
The ranking process in UBMS Analytics defines the priority accorded to a particular bridge from a set of bridges that need rehabilitation intervention. The impact of LCCA on the ranking process is seen by an actual example for a set of bridges numbered from 1 to 14 for ease of understanding. The ranking when LCCA analytics is not applied and ranking is based on a conventional process which is determined by Wsum is different when the ranking is subjected to analytics using LCCA where the impact of socio-economic parameters and the importance of the type of road are accounted into the ranking process. The below two tables signify the impact of LCCA on the ranking process.
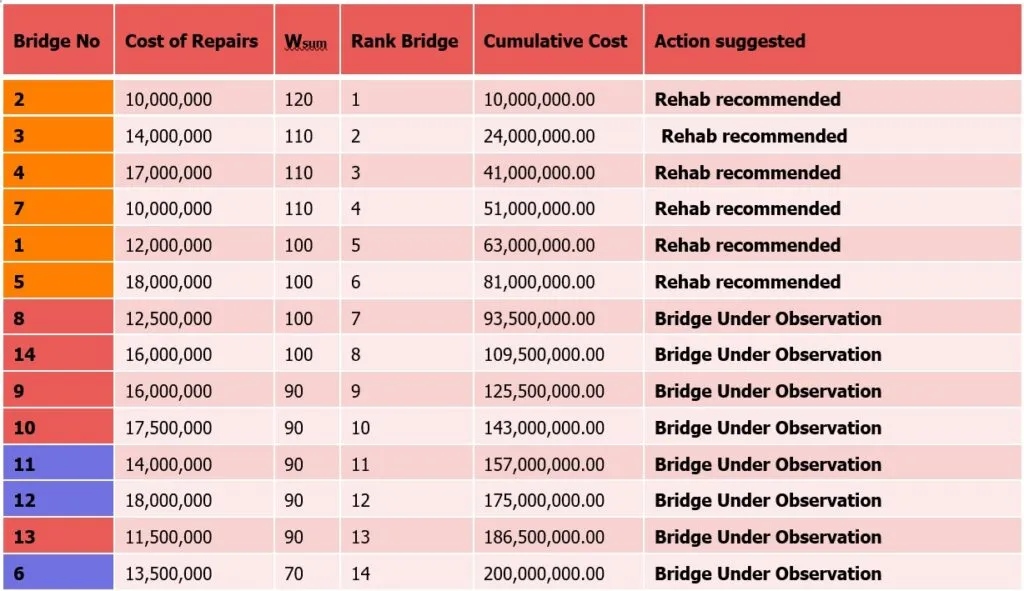
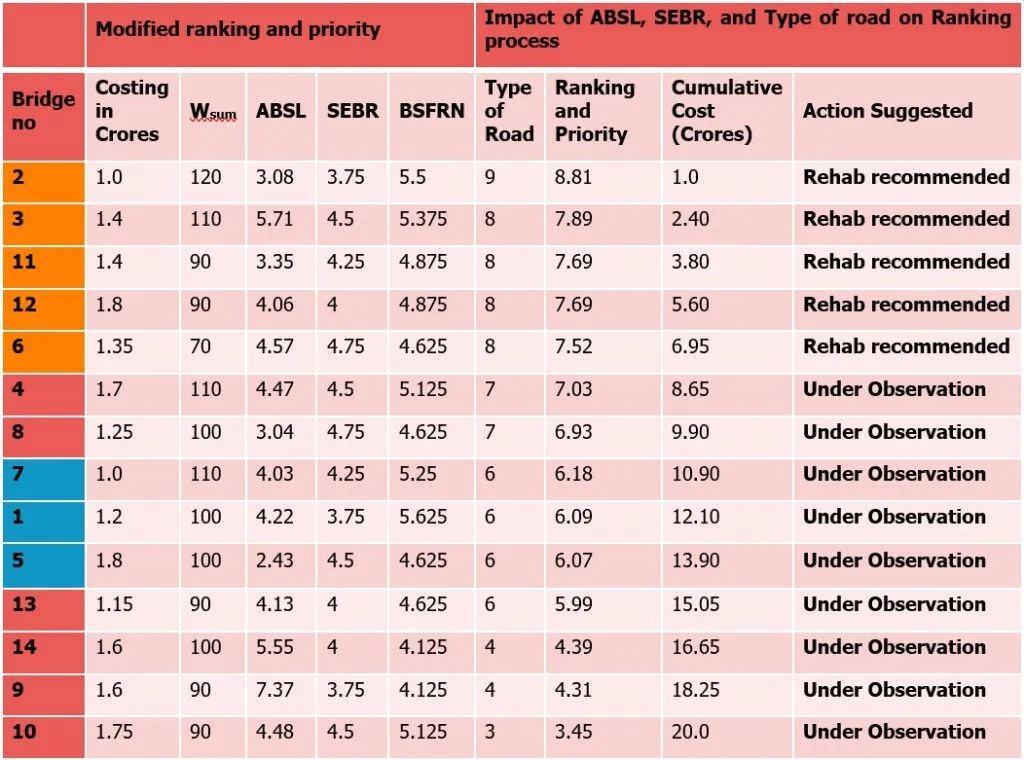
- CONCLUSION
Bridge management analytics in UBMS ensures that the various goals of sustainability and economy are fulfilled. The Bridge Management Analytics that ensure this includes the application of realistic and factual data for deterioration model generation, the inclusion of Socio-economic parameters to evaluate the benefits [Direct and Indirect included] that accrue to the society due to the presence of bridge infrastructure, application of LCCA tool to evaluate the life cycle costing that are then applied to the decision-making process.
UBMS has data collected for various socio-economic parameters. This data is collated in the form of ratings for Socio-Economic parameters. The application of economic gain or loss to these ratings ensures the evaluation of intangible benefits or costs that accrue from Bridge infrastructure. The application of the LCCA tool ensures that the intangible gains due to bridge infrastructure are taken note of in the decision-making process. This analysis also ensures Sustainability goals that bridge infrastructure meets the needs of the present without compromising the ability of the future generation to meet their own needs. A sustainable path should maintain the balance between the three important aspects of Social, Economic, and Environmental considerations. Sustainability ensures supporting the natural, social and economic system upon which we depend now and the future generations will depend in the future. This shall ensure net gain in the three systems without adversely affecting them. One needs to provide the required level of service in the most cost-effective way within the parameters of Sustainability.
REFERENCES
- BRIDGE LIFE CYCLE COST ANALYSIS 2020 JETIR MAY 2020, VOLUME 7, ISSUE 5.
- BERTHA MARIA BATISTA SANTOS, SIMPLIFIED MODEL OF ROAD-USER COSTS FOR PORTUGUESE HIGHWAYS, 07 OCTOBER 2015.
- NATIONAL COOPERATIVE HIGHWAY RESEARCH PROGRAM, NCHRP REPORT 713 ESTIMATING LIFE EXPECTANCIES OF HIGHWAY ASSETS, VOLUME 1.
- NATIONAL COOPERATIVE HIGHWAY RESEARCH PROGRAM NCHRP REPORT 483, BRIDGE LIFE-CYCLE COST ANALYSIS.
- TOWARDS PERFORMANCE-BASED DYNAMIC & REAL TIME BRIDGE MANAGEMENT, BY SACHIDANAND JOSHI & OTHERS AUGUST 25, 2022, CONSTRO-FACILITATOR.
- MMM GROUP, PRIMER ON THE SUSTAINABILITY CONSIDERATIONS FOR BRIDGES, TRANSPORTATION ASSOCIATION OF CANADA (TAC), OCTOBER 2015.
- BUILDING AND MAINTAINING BRIDGES TO BE SUSTAINABLE WIJE ARIYARATNE V. PONNAMBALAM, SUSTAINABLE BRIDGES: THE THREAD OF SOCIETY, ROADS AND TRAFFIC AUTHORITY OF NSW, AUSTRALIA.
- ROLE OF THE SOCIAL DIMENSION IN THE SUSTAINABILITY-ORIENTED MAINTENANCE OPTIMIZATION OF BRIDGES IN COASTAL ENVIRONMENTS, IGNACIO J. NAVARRO1, WIT TRANSACTIONS ON THE BUILT ENVIRONMENT, VOL 196, © 2020 WIT PRESS.
- MARKOW ET. AL, NCHRP SYNTHESIS 397: BRIDGE MANAGEMENT SYSTEMS FOR TRANSPORTATION AGENCY DECISION MAKING, TRANSPORTATION RESEARCH BOARD (TRB), MAY 28, 2014.
- UPPER HUNTER SHIRE COUNCIL, BRIDGES ASSET MANAGEMENT PLAN, DVC 2006.
- LEIVA MALDONADO, M. D. (2019). LIFE-CYCLE COST ANALYSIS FOR SHORT- AND MEDIUM-SPAN BRIDGES (JOINT TRANSPORTATION RESEARCH PROGRAM PUBLICATION NO. FHWA/IN/JTRP-2019/09).
- DR. KAAN OZBAY ET. AL, GUIDELINES FOR LIFE CYCLE COST ANALYSIS, JULY 2003, FHWA-NJ-2003-012.
- STEFANO SACCONI ET. AL, LIFE-CYCLE COST ANALYSIS OF BRIDGES SUBJECTED TO FATIGUE DAMAGE, JOURNAL OF INFRASTRUCTURE PRESERVATION AND RESILIENCE, PUBLISHED: 11 OCTOBER 2021.
- MASOUD DEHGHANI CHAMPIRI ET.AL. ASSESSING DISTRESS CAUSE AND ESTIMATING EVALUATION INDEX FOR MARINE CONCRETE STRUCTURES, AMERICAN JOURNAL OF CIVIL ENGINEERING AND ARCHITECTURE, 2016, VOL. 4, NO. 4, 142-152.
- ANDREAS LEEMANN ET, AL. AN ATTEMPT TO VALIDATE THE ULTRA-ACCELERATED MICROBAR AND THE CONCRETE PERFORMANCE TEST WITH THE DEGREE OF AAR-INDUCED DAMAGE OBSERVED IN CONCRETE STRUCTURES, RESEARCHGATE, JULY 2013.
- N. JAMALUDDIN ET. AL, FORENSIC BUILDING: DETERIORATION AND DEFECT IN CONCRETE STRUCTURES, MATEC WEB OF CONFERENCES 103, 02016 (2017).
- HANDBOOK FOR IMPLEMENTING IBMS/UBMS, SACHIDANAND JOSHI AND OTHERS, 2019-20.
- MICHAEL RAUPACH TILL BÜTTNER, CONCRETE REPAIR- DIAGNOSIS, DESIGN, PRINCIPLES AND PRACTICE, EN 1504.
Authored by:
Sachidanand Joshi Head- UBMS Research Group (URG),
Atharvi Thorat, Harshali Dehadray, Mayuri Tundalwar – Research Engineers- URG